Bob McDonald
Books by Bob McDonald

Just Say Yes
A Memoir

The Future Is Now
Solving the Climate Crisis with Today's Technologies

On Our Nature Walk Read-Along
Our First Talk About Our Impact on the Environment

On Our Nature Walk
Our First Talk About Our Impact on the Environment

An Earthling's Guide to Outer Space
Everything You Ever Wanted to Know About Black Holes, Dwarf Planets, Aliens, and More

Canadian Spacewalkers
Hadfield, MacLean and Williams Remember the Ultimate High Adventure

The Quirks & Quarks Guide to Space
42 Questions (and Answers) About Life, the Universe, and Everything
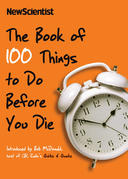
Book of 100 Things to Do Before You /tp

The Quirks & Quarks Question Book
101 Answers to Listeners' Questions